High-Resolution 3D Bioprinter Leases to Fit Every Need
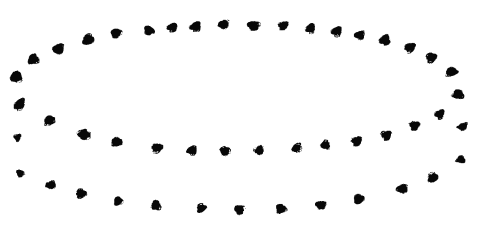
Founder-Friendly Leases
Our lease agreements are founder-friendly and flexible, helping you preserve working capital, strengthen the cash flow of your business, and keep business credit lines open for expansions, staffing, and other crucial operational expenses and business development opportunities.
2-5 Year Lease Lengths
Leases range from 2 to 5 years. Length will depend on several factors, including how long you want to use the equipment, equipment type, and your company’s financial position. These are standard factors leasing companies consider and help us tailor a lease agreement to fit your needs.
Your Choice of Manufacturer
We don’t carry an inventory. This means you’re not limited to a specific set of manufacturers. Instead, you can pick the equipment that aligns with your business goals and preferences. We’ll work with the manufacturer of your choice to get the equipment in your facility as quickly as possible.
Maintenance & Repair Coverage
Bundle preventive maintenance and repair coverage with your lease agreement. You can spread those payments over time. Easily maintain your equipment, minimize the chances something will break down, repair instrumentation quickly, and simplify your payment processes.
End-of-Lease Options
At the end of your lease, you have multiple options. You can either renew the lease at a significantly lower price, purchase the machine outright based on the fair market value of the original pricing, or call it a day and we’ll come the pick up the equipment for you free of charge.
No Loan-Like Terms
Our leases do not include loan-like terms, which can be restrictive or harmful in certain situations. We do not require debt covenants, IP pledges, collateral, or equity participation. Our goal is to maximize your flexibility. When you lease with us, you’re collaborating with a true business partner.
In-House Underwriting Process
Our underwriting is done in-house. You can expect quicker turnaround, allowing you respond to your equipment needs as they arise. We require less documentation than traditional lenders and financiers and can get the equipment you need in operation more quickly.